Study by U of T researchers reveals how bacterial toxins evolve to cause new illnesses
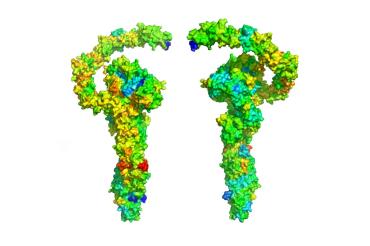
Published: July 2, 2020
The coronavirus pandemic is a daily reminder of the far-reaching consequences of a pathogen’s successful invasion of human cells. And, as a new University of Toronto study on bacterial toxins shows, it does not take much for these encounters to turn deadly.
The research found that two almost identical bacterial toxins cause distinct illnesses – diarrhea and fatal toxic shock syndrome – by binding unrelated human receptors. It also highlights a mechanism by which pathogens have evolved distinct receptor preferences to infect different organs.
“I always think of bacterial toxins as fascinating machines of death in how they find new ways to enter host tissue,” says Mikko Taipale, an assistant professor of molecular genetics at the Donnelly Centre for Cellular and Biomolecular Research.
Taipale co-led the study, published recently in the journal Cell, with Roman Melnyk and Jean-Philippe Julien, both senior scientists at the Hospital for Sick Children and faculty members in U of T’s department of biochemistry.
Many are familiar with Clostridium difficile, a gut-dwelling bacterium that can cause diarrhea. Lesser known is its close relative, Paeniclostridium sordellii, which also lives in the gut and in the female reproductive tract. Infections are rare but fatal and can occur when the bacterial toxin escapes into the bloodstream, during birth for example, and spreads into the lungs and other organs.
Both species are thought to be part of the microbiome, the body’s resident bacteria, but it’s not clear why they harm some people and not others.
The toxin released by C. difficile acts through Frizzled receptor proteins, which play a role in tissue regeneration. Yet, while P. sordellii produces a similar toxin, it does not bind in the same way – so the researchers set out to investigate.
They took an unbiased approach by systematically switching off every gene in human cells and exposing them to the P. sordellii toxin. Cells that survived turned out to lack genes encoding cell surface proteins called semaphorins, and other experiments confirmed that two members of this class, Semaphorin6A and Semaphorin6B, are indeed the receptors for the toxin. Both receptors are present in the lungs, as expected, though their role there remains unclear.
Knowing the receptor opens the door to finding treatment. The researchers were able to halt infection in mice by co-injecting the toxin with purified semaphorin fragments, which bound and neutralized the toxin before it could reach the real receptors.
But the finding led to more surprises.
Like Frizzled, semaphorins play important roles in the body – most notably in developing the nervous system, where they help guide projecting nerve fibers. Even more surprising was that it binds a receptor with no structural resemblance to Frizzled.
“Here we have two toxins that are so similar to each other, but they use completely different receptors,” says Taipale. “We did not expect to find that.”
The reason is a tiny difference between C. difficile and P. sordellii – in particular, the surface though which both toxins contact their receptors, as revealed by cryo electron microscopy. Each toxin protein is composed of about 2,500 amino-acids and the researchers were able to pinpoint those that directly engage with the receptor. Swapping a mere 15 of these amino acids between the two toxins was sufficient to switch receptor preference. In other words, they created a P. sordellii toxin that targeted Frizzled and vice versa.
“We were floored when we saw that the toxins shared a surface that each evolved to uniquely interact with distinct cells,” says Julien.
It appears that, while the rest of the toxin is under strong evolutionary pressure to remain unchanged, the receptor-binding surface is free from such constraints. This allows toxins to evolve into variants that bind new receptors to invade other tissues and hosts.
Receptor switching is not unique to bacteria, however. SARS-CoV-2 and coronavirus strains that cause common cold use the same part of the now famous spike protein to bind to diverse receptors, which might explain differences in disease severity.
“This is a nice example of how viruses and bacteria – from completely different domains of life – have found similar molecular tactics to change their receptor targets in human cells,” says Taipale.
“It also reminds us how much cool biology one can find in the microbial world.”
The research was supported by the CIFAR Azrieli Global Scholar program, the Ontario Early Researcher Awards program and the Canada Research Chairs program.